The Pursuit of a Comprehensive Theory of Gravity
Written on
Chapter 1: The Significance of Gravity
Without the force of gravity, the night sky would appear devoid of celestial bodies. Stars, galaxies, moons, and planets owe their existence to gravity, which binds them together. This force is also responsible for the formation of our Sun and Earth, having pulled together dispersed atoms to create the universe we observe today.
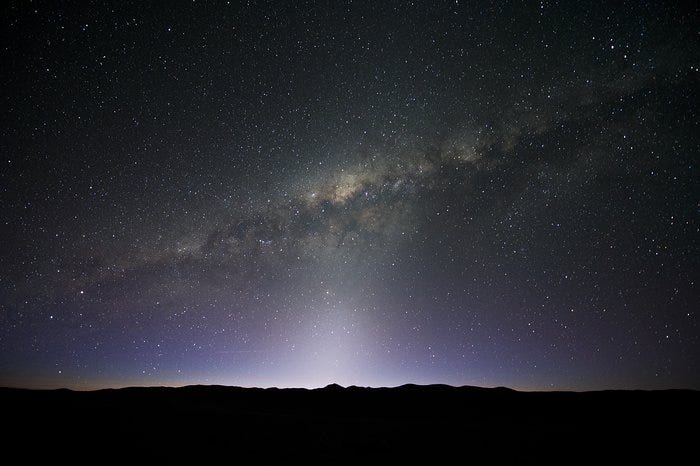
The beauty of the night sky is a testament to gravity's role. Credit to ESO.
Gravity has been central to humanity's quest to comprehend the nature of reality, from Newton’s universal law to Einstein’s relativity. In contemporary science, gravity remains pivotal, potentially holding the answer to what could be the ultimate theory of physics.
Despite its profound influence, gravity is surprisingly the weakest of the four fundamental forces. This is easily demonstrated; for instance, a small fridge magnet can effortlessly lift a pin, overcoming the gravitational pull of an entire planet.
This weakness allows gravity to be largely disregarded at atomic and molecular levels. It is only when examining vast entities—such as planets, stars, and galaxies—that gravity becomes significant. While the other fundamental forces diminish over short distances, gravity's influence extends across galaxies.
Humanity has recognized gravity's effects since ancient times. The simple observation that objects fall when dropped is universally understood and would have been evident even in prehistoric eras. Civilizations in Greece and India developed a rudimentary understanding of gravity, acknowledging that falling objects accelerate, yet they could not apply these concepts to the larger cosmos.
It was not until the Renaissance, during the time of Galileo, Kepler, and Newton, that a scientific exploration of gravity took shape. Galileo's experiments revealed the surprising reality that all objects, regardless of weight, fall at the same rate. Kepler, utilizing planetary observations, formulated laws describing the planets' motions around the Sun.
In 1687, Isaac Newton published "Philosophiæ Naturalis Principia Mathematica," encapsulating his three laws of motion and the universal law of gravitation. For the first time, gravity was articulated mathematically, allowing Newton to demonstrate that Kepler’s laws stemmed from a fundamental equation governing gravity. This revelation established gravity as the force shaping the universe, orchestrating the movements of stars and planets, and influencing seasonal changes, tides, and even falling apples.
In the following two centuries, scientists expanded upon Newton’s principles, giving rise to Classical Physics. This new framework proved exceptionally successful in describing and predicting natural phenomena. Astronomers, noticing inconsistencies in Uranus’s orbit, used classical physics to predict the existence of another planet beyond it, leading to Neptune's discovery in 1846.
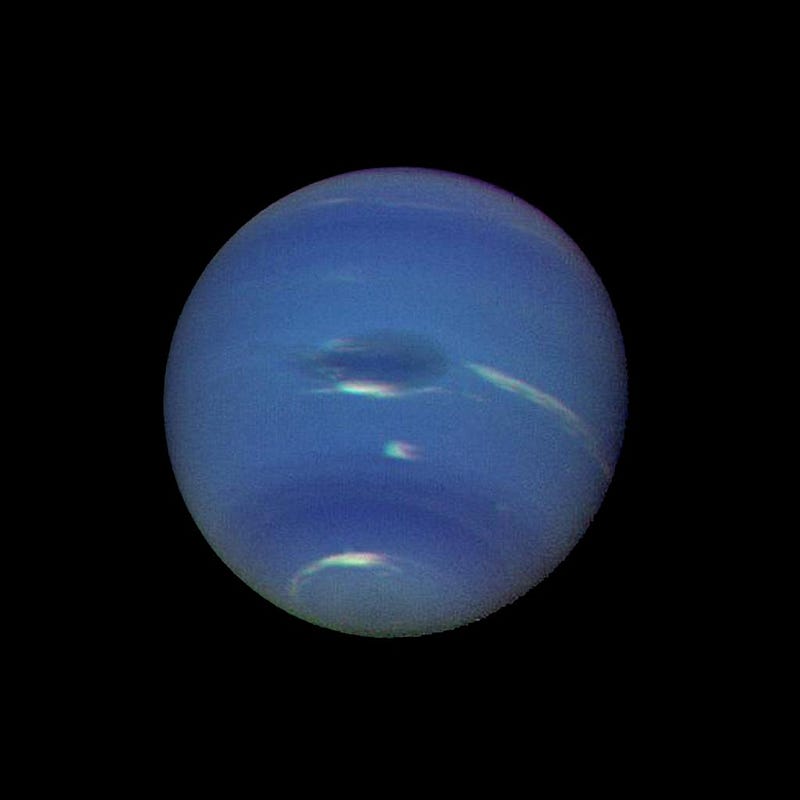
Neptune's existence was predicted based on Uranus's orbital deviations. Credit to NASA.
Despite these advancements, challenges emerged in our understanding of gravity. The orbit of Mercury deviated from predictions, prompting astronomers to search for another planet to account for this anomaly. Additionally, while scientists could explain gravitational effects, the question of how distant stars and planets exert force on Earth remained unresolved.
Addressing these mysteries necessitated a paradigm shift in physics, which occurred in the early twentieth century. Classical Physics was rapidly overshadowed by two revolutionary theories: Quantum Physics and Relativity. Quantum theory primarily addressed phenomena at microscopic scales, often neglecting gravity, while relativity became closely intertwined with gravitational concepts.
Einstein's renowned special theory of relativity focused on the speed of light and the behavior of objects moving near that speed. Initially, it had little relevance to gravity. However, Einstein later developed a more comprehensive framework known as the General Theory of Relativity, which portrayed gravity more accurately than Newton's approach. This theory not only clarified Mercury's orbital issues but also predicted the existence of exotic entities such as black holes, wormholes, and even the potential for time travel.
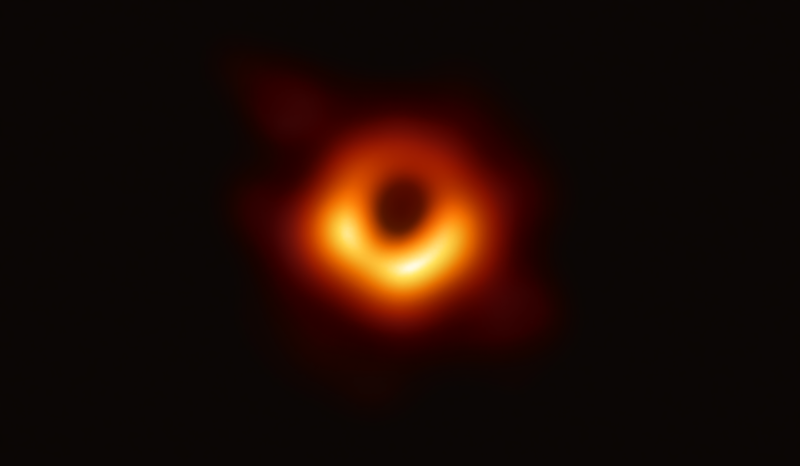
The first image of a black hole validates Einstein’s predictions. Credit to EHT.
The general theory of relativity is a remarkably elegant framework. In classical physics, the concepts of matter, motion, space, and time are considered separately. Space serves as a backdrop where matter interacts, while time merely ticks away. However, in relativity, these concepts merge. Space and time unite into a single entity known as spacetime. The presence of matter warps spacetime, and as matter traverses both space and time, these distortions influence the movement of both matter and light.
Arthur Eddington confirmed the predictions of general relativity in 1919. Further studies revealed solutions suggesting the existence of black holes—immense objects that warp space so intensely that light cannot escape. Initially regarded as mere mathematical abstractions, the concept of black holes gradually gained acceptance over the decades. Additional solutions to Einstein’s equations have proposed the possibility of peculiar structures such as wormholes, which might connect distant regions of space via higher-dimensional pathways or even allow for closed time loops.
Nonetheless, unresolved challenges regarding gravity persisted. Our understanding of the universe's scale transformed dramatically in the early twentieth century with the identification of other galaxies. However, observations indicated that these galaxies did not rotate at speeds predicted by gravitational theories. To address this discrepancy, physicists posited the existence of "Dark Matter," theorized to be a nearly invisible particle contributing extra mass to galaxies. Despite extensive searches, the nature of dark matter remains elusive, prompting some scientists to explore alternative solutions, including modifications to gravitational laws.
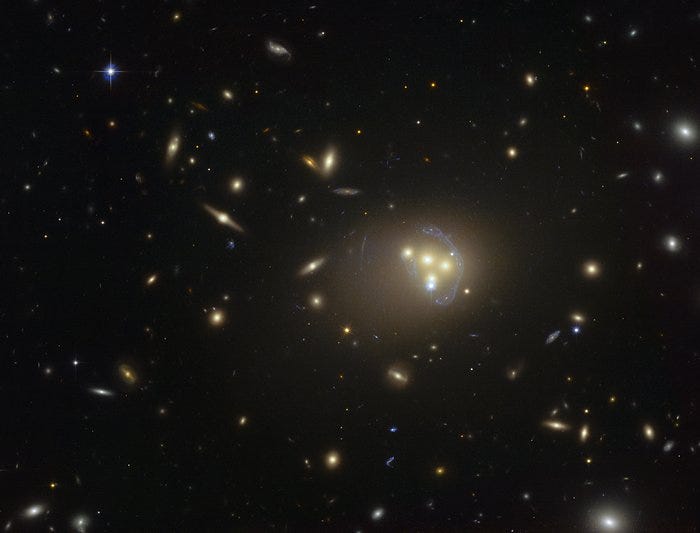
Numerous galaxies in the Abell 3827 cluster, with motion explained by dark matter. Credit to ESO.
Throughout the 20th century, three of the four fundamental forces of nature were articulated using quantum physics. In this framework, each force is associated with a specific particle: the photon for electromagnetism, and for the two nuclear forces (the Strong and Weak Nuclear Forces), particles known as gluons and the W and Z bosons.
It stands to reason that the fourth fundamental force, gravity, would similarly have an associated quantum particle—the graviton. Although theoretically well-defined, the graviton is incredibly challenging to detect. Designing a detector capable of identifying the graviton is daunting, and many scientists believe constructing such a device on Earth is unfeasible. Additionally, integrating the graviton within the broader mathematical framework of quantum physics presents significant hurdles.
Developing a quantum theory of gravity remains one of physics' foremost challenges. The quest for quantum gravity has spawned numerous theories, including string theory, M-theory, quantum loop gravity, twister theory, and M8 theory. Theoretical physicists have proposed a multitude of ideas for reconciling gravity with quantum mechanics, yet determining which, if any, is accurate has proven elusive.
The fundamental weakness of gravitational force poses a significant obstacle. Its effects are generally undetectable at the quantum level, only becoming apparent in extreme cosmic conditions—such as during the big bang or within black holes—where quantum gravity might manifest. However, black holes are inherently unobservable, and we cannot peer back to the universe's earliest moments when quantum gravity may have been present.
Consequently, the ultimate theory of gravity remains out of reach for now. Physicists will undoubtedly continue to construct theoretical models of quantum gravity, yet without a means to empirically test these theories, substantial progress is unlikely. In the absence of a quantum gravity theory, many of the universe’s most puzzling and enigmatic phenomena will remain beyond our comprehension.
Chapter 2: The Search for the Ultimate Theory
The first video titled "The Quest for the Ultimate Theory" explores the ongoing search for a unified understanding of the forces of nature and how gravity plays a crucial role in this quest.
The second video, "Loose Ends: String Theory and the Quest for the Ultimate Theory," delves into string theory's implications and its connection to the fundamental forces, including gravity.